Influencia del ángulo de fibra y caracterización de la tenacidad de materiales compuestos helicoidales de fibra discontinua bioinspirados producidos mediante fabricación aditiva
Fiber Angle influence and Toughness characterization of bioinspired discontinuous fiber helicoids composite materials produced via additive manufacturing
Barra lateral del artículo
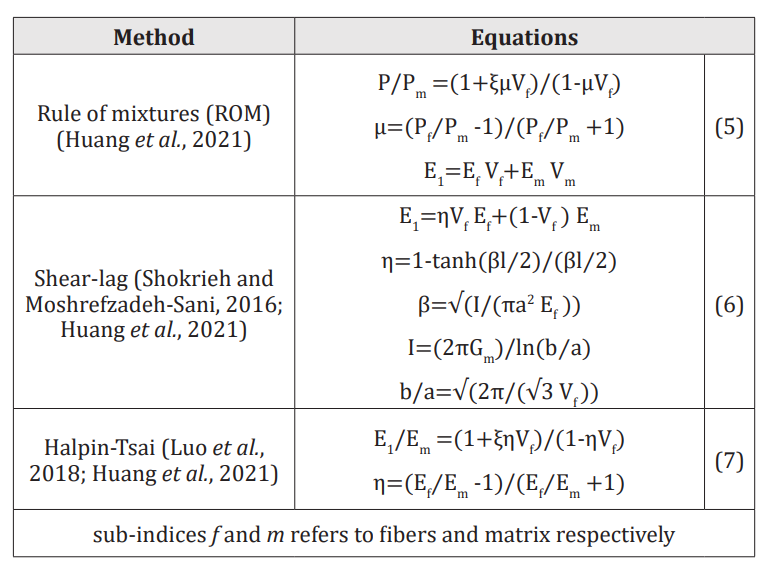
Términos de la licencia (VER)

Esta obra está bajo una licencia internacional Creative Commons Atribución-NoComercial-SinDerivadas 4.0.
Declaración del copyright
Los autores ceden en exclusiva a la Universidad EIA, con facultad de cesión a terceros, todos los derechos de explotación que deriven de los trabajos que sean aceptados para su publicación en la Revista EIA, así como en cualquier producto derivados de la misma y, en particular, los de reproducción, distribución, comunicación pública (incluida la puesta a disposición interactiva) y transformación (incluidas la adaptación, la modificación y, en su caso, la traducción), para todas las modalidades de explotación (a título enunciativo y no limitativo: en formato papel, electrónico, on-line, soporte informático o audiovisual, así como en cualquier otro formato, incluso con finalidad promocional o publicitaria y/o para la realización de productos derivados), para un ámbito territorial mundial y para toda la duración legal de los derechos prevista en el vigente texto difundido de la Ley de Propiedad Intelectual. Esta cesión la realizarán los autores sin derecho a ningún tipo de remuneración o indemnización.
La autorización conferida a la Revista EIA estará vigente a partir de la fecha en que se incluye en el volumen y número respectivo en el Sistema Open Journal Systems de la Revista EIA, así como en las diferentes bases e índices de datos en que se encuentra indexada la publicación.
Todos los contenidos de la Revista EIA, están publicados bajo la Licencia Creative Commons Atribución-NoComercial-NoDerivativa 4.0 Internacional
Licencia
Esta obra está bajo una Licencia Creative Commons Atribución-NoComercial-NoDerivativa 4.0 Internacional
Contenido principal del artículo
Resumen
Durante milenios la naturaleza ha producido materiales compuestos con excelentes propiedades mecánicas frente a las bajas propiedades de sus materiales base y ha conseguido obtener una buena compatibilidad entre rigidez y tenacidad; como resultado, son una buena fuente de inspiración para la optimización de materiales para aplicaciones, como el aumento de la tenacidad y la resistencia al daño, algo que es difícil en los materiales de ingeniería convencionales. En la actualidad se identifican ocho elementos estructurales en los materiales biológicos: fibroso, helicoidal, degradado, estratificado, tubular, celular, sutura y superpuesto. Las estructuras helicoidales consisten en pilas de fibras ordenadas que forman capas que giran en un ángulo de inclinación constante. Estos incluyen estructuras de madera contrachapada y Bouligand. Las estructuras de Bouligand consisten en una disposición de laminados fibrosos que completa un giro de 180° y proporciona a algunos materiales biológicos mayor resistencia y tenacidad en múltiples direcciones y una tenacidad a la fractura excepcionalmente alta. La mecánica clásica de materiales compuestos proporciona algunas aproximaciones de modelos constitutivos para este tipo de materiales, pero aún necesitan ser estudiados y probados para comprender adecuadamente su comportamiento. El moldeo por inyección, el moldeo por compresión, la disposición manual, el moldeo por transferencia de resina, el bobinado de filamentos, la pultrusión y la colocación automatizada de fibras son solo algunos de los métodos tradicionales utilizados para fabricar compuestos de polímeros reforzados con fibras (FRPC). Sin embargo, estas técnicas de fabricación tradicionales tienen una restricción en la alineación de fibra específica y exigen moldes, troqueles o máscaras litográficas costosos. La fabricación aditiva tiene el potencial de reemplazar muchos procesos de fabricación convencionales debido a su capacidad para crear geometrías complejas con propiedades de materiales personalizables y emplear varios materiales simultáneamente, entre otras cosas. El modelado por deposición fundida (FDM) es la técnica de fabricación aditiva más utilizada para la fabricación de FRPC debido a su bajo costo, bajo consumo de energía, consumo de material y simplicidad de operación. Este trabajo presenta modelos tridimensionales que imitan las estructuras de Bouligand girando el ángulo de inclinación de las capas y se realizó una comparación de modelos analíticos. Los especímenes fueron fabricados usando la técnica FDM. Se utilizó poliuretano termoplástico (TPU) para la matriz y ácido poliláctico (PLA) para las fibras. Se utilizaron pruebas de tracción para caracterizar mecánicamente tanto las materias primas como los compuestos fabricados para examinar el impacto del ángulo helicoidal y la contribución de la matriz y los materiales de fibra a la rigidez y tenacidad del compuesto. Los experimentos y análisis revelaron que los altos ángulos de rotación mejoran la rigidez, la resistencia y la tenacidad del compuesto.
Descargas
Detalles del artículo
Referencias (VER)
Arias, L. S. and Vanegas, L. (2004) ‘Falla de los materiales compuestos laminados’, Scientia et Technica, (25), pp. 113–118.
Autodesk (no date) Inventor: Poderoso software de diseño mecánico para tus ideas más ambiciosas.
Barthelat, F. et al. (2007) ‘On the mechanics of mother-of-pearl: A key feature in the material hierarchical structure’, Journal of the Mechanics and Physics of Solids, 55(2), pp. 306–337. doi: 10.1016/j.jmps.2006.07.007.
Barthelat, F., Yin, Z. and Buehler, M. J. (2016) ‘Structure and mechanics of interfaces in biological materials’, Nature Reviews Materials, 1(16007), pp. 1–16. doi: 10.1038/natrevmats.2016.7.
Bruet, B. J. F. et al. (2008) ‘Materials design principles of ancient fish armour.’, Nature materials. Nature Publishing Group, 7(9), pp. 748–56. doi: 10.1038/nmat2231.
Carvajal Loaiza, M. J. et al. (2020) ‘Influencia de la posición de impresión y la densidad de relleno en las propiedades mecánicas de probetas fabricadas en ABS’, Revista Ingenierías Universidad de Medellín. Universidad de Medellín, 19(37), pp. 179–193. doi: 10.22395/RIUM.V19N37A9.
Chen, P.-Y. et al. (2008) ‘Structure and mechanical properties of crab exoskeletons’, Acta Biomaterialia, 4(3), pp. 587–596. doi: 10.1016/j.actbio.2007.12.010.
Chen, P.-Y. et al. (2012) ‘Predation versus protection: Fish teeth and scales evaluated by nanoindentation’, Journal of Materials Research, 27(1), pp. 100–112.
Chen, S.-M. et al. (2022) ‘Biomimetic discontinuous Bouligand structural design enables high-performance nanocomposites’, Matter. Elsevier Inc., pp. 1–15. doi: 10.1016/j.matt.2022.02.023.
Chen, S. M. et al. (2018) ‘Biomimetic twisted plywood structural materials’, National Science Review, 5(5). doi: 10.1093/nsr/nwy080.
Cheng, L. et al. (2011) ‘Mechanical behavior of bio-inspired laminated composites’, Composites Part A, 42, pp. 211–220. doi: 10.1016/j.compositesa.2010.11.009.
Cheng, L., Wang, L. and Karlsson, A. M. (2008) ‘Image analyses of two crustacean exoskeletons and implications of the exoskeletal microstructure on the mechanical behavior’, Journal materials research society, 23(11), pp. 2854–2872. doi: 10.1557/JMR.2008.0375.
‘Engineering for ENERGY INFRASTRUCTURE 4.0 TECHNOLOGY VITAL ENGINEERING Facultad de Ingeniería’ (no date).
Fang, Z. et al. (2014) ‘Hierarchical structure and cytocompatibility of fish scales from Carassius auratus’, Materials Science and Engineering: C, 43, pp. 145–152. doi: 10.1016/j.msec.2014.07.015.
Gao, W. et al. (2015) ‘The status, challenges, and future of additive manufacturing in engineering’, Computer-Aided Design, 69, pp. 65–89. doi: 10.1016/j.cad.2015.04.001.
Gil-Duran, S., Arola, D. and Ossa, E. A. (2015) ‘Effect of chemical composition and microstructure on the mechanical behavior of fish scales from Megalops Atlanticus’, Journal of the Mechanical Behavior of Biomedical Materials, 56, pp. 134–145. doi: 10.1016/j.jmbbm.2015.11.028.
Grunenfelder, L. K. et al. (2014) ‘Bio-inspired impact-resistant composites.’, Acta biomaterialia, 10(9), pp. 3997–4008. doi: 10.1016/j.actbio.2014.03.022.
Guarín-Zapata, N. et al. (2015) ‘Shear wave filtering in naturally-occurring Bouligand structures.’, Acta biomaterialia, 23, pp. 11–20. doi: 10.1016/j.actbio.2015.04.039.
Hu, C. et al. (2019) ‘Bioinspired surface modification of orthopedic implants for bone tissue engineering’, Biomaterials. Elsevier, 219(June), p. 119366. doi: 10.1016/j.biomaterials.2019.119366.
Huang, Y. et al. (2015) ‘Additive manufacturing: Current state, future potential, gaps and needs, and recommendations’, Journal of Manufacturing Science and Engineering, Transactions of the ASME. American Society of Mechanical Engineers (ASME), 137(1).
doi: 10.1115/1.4028725.
Huang, Z.-M. et al. (2021) ‘Tensile Strength Prediction of Short Fiber Reinforced Composites’, Materials, 14(2708), pp. 1–22. doi: 10.3390/ma14112708.
Ikoma, T. et al. (2003) ‘Microstructure, mechanical, and biomimetic properties of fish scales from Pagrus major’, Journal of Structural Biology. Academic Press, 142(3), pp. 327–333. doi: 10.1016/S1047-8477(03)00053-4.
Ji, B. and Gao, H. (2004) ‘Mechanical properties of nanostructure of biological materials’, Journal of the Mechanics and Physics of Solids, 52(9), pp. 1963–1990. doi: 10.1016/j.jmps.2004.03.006.
Kienzler, R., Ott, I. and Altenbach, H. (eds) (2004) Theories of Plates and Shells. Springer Berlin Heidelberg (Lecture Notes in Applied and Computational Mechanics). doi: 10.1007/978-3-540-39905-6.
Körbelin, J. et al. (2021) ‘Damage tolerance and notch sensitivity of bio-inspired thinply Bouligand structures’, Composites Part C: Open Access. Elsevier, 5, pp. 1–14. doi: 10.1016/j.jcomc.2021.100146.
Li, J. et al. (2022) ‘Spider Silk-Inspired Artificial Fibers’, Advanced Science. John Wiley and Sons Inc, 9(5). doi: 10.1002/ADVS.202103965.
Liu, J. L. et al. (2022) ‘Effects of inter-ply mismatch angle on interlaminar properties and their influence in numerical simulations’, Composites Part A: Applied Science and Manufacturing. Elsevier Ltd, 154. doi: 10.1016/j.compositesa.2021.106795.
Luo, Z. et al. (2018) ‘Modified rule of mixtures and Halpin-Tsai model for prediction of tensile strength of micron-sized reinforced composites and Young’s modulus of multiscale reinforced composites for direct extrusion fabrication’, Research Article Advances in
Mechanical Engineering, 10(7), pp. 1–10. doi: 10.1177/1687814018785286.
Meyers, M. A. et al. (2008) ‘Biological materials: Structure and mechanical properties’, Progress in Materials Science, 53(1), pp. 1–206. doi: 10.1016/j.pmatsci.2007.05.002.
Meyers, M. A. and Hodge, A. M. (2008) Advances in Biological Materials and Biomaterials Science.
Murcia, S. et al. (2016) ‘Effects of polar solvents on the mechanical behavior of fish scales’, Materials Science and Engineering: C. Elsevier, 61, pp. 23–31. doi: 10.1016/J. MSEC.2015.12.007.
Ni, J. et al. (2021) ‘Strong fatigue-resistant nanofibrous hydrogels inspired by lobster underbelly’, Matter, 4(6), pp. 1919–1934. doi: 10.1016/j.matt.2021.03.023.
Ouyang, W. et al. (2021) ‘Identifying optimal rotating pitch angles in composites with Bouligand structure’, Composites Communications. Elsevier Ltd, 23, p. 100602. doi: 10.1016/j.coco.2020.100602.
Pérez, M. A. and Sánchez, M. (2014) Fundamentos de la mecánica de los materiales compuestos, Aplicaciones avanzadas de los materiales compuestos en la obra civil y la edificación. doi: 10.3926/oms.200.
Reddy, J. N. (1999) ‘Theory and analysis of laminated composite plates’, in Mechanics of Composite Materials and Structures. Kluwer Academic Publishers, pp. 1–79. doi: 10.1007/978-94-011-4489-6_1.
Salinas, C. and Kisailus, D. (2013) ‘Fracture mitigation strategies in gastropod shells’, JOM, 65(4). doi: 10.1007/s11837-013-0570-y.
Shokrieh, M. M. and Moshrefzadeh-Sani, H. (2016) ‘On the constant parameters of Halpin-Tsai equation’, Polymer. Elsevier Ltd, 106, pp. 14–20. doi: 10.1016/j.polymer.2016.10.049.
Suksangpanya, N. et al. (2018) ‘Crack twisting and toughening strategies in Bouligand architectures’, International Journal of Solids and Structures. Pergamon. doi: 10.1016/J.IJSOLSTR.2018.06.004.
Torres, F. G. et al. (2008) ‘Characterization of the nanocomposite laminate structure occurring in fish scales from Arapaima Gigas’, Materials Science and Engineering: C, 28(8), pp. 1276–1283. doi: 10.1016/j.msec.2007.12.001.
Ultimaker (no date) “Ultimaker Cura: software de impresión 3D potente y fácil de usar”.
Wang, D. et al. (2020) ‘Fiber reorientation in hybrid helicoidal composites’, Journal of the Mechanical Behavior of Biomedical Materials. Elsevier Ltd, 110(June), p. 103914. doi: 10.1016/j.jmbbm.2020.103914.
Weaver, J. C. et al. (2012) ‘The stomatopod dactyl club: A formidable damage-tolerant biological hammer’, Science, 336(6086), pp. 1275–1280. doi: 10.1126/science.1218764.
Wu, K. et al. (2020) ‘Discontinuous fibrous Bouligand architecture enabling formidable fracture resistance with crack orientation insensitivity’, Proceedings of the National Academy of Sciences of the United States of America, 27(117), p. 8. doi: 10.1073/
pnas.2000639117/-/DCSupplemental.
Yang, F., Yi, F. and Xie, W. (2021) ‘The role of ply angle in interlaminar delamination properties of CFRP laminates’, Mechanics of Materials. Elsevier, 160, p. 103928. doi: 10.1016/J.MECHMAT.2021.103928.
Yang, W. et al. (2014) ‘Protective role of Arapaima gigas fish scales: structure and mechanical behavior.’, Acta biomaterialia, 10(8), pp. 3599–614. doi: 10.1016/j.actbio.2014.04.009.
Yang, W. et al. (2019) ‘Arapaima Fish Scale: One of the Toughest Flexible Biological Materials’, Matter. Elsevier Inc., 1(6), pp. 1557–1566. doi: 10.1016/j.matt.2019.09.014.
Yin, S. et al. (2020) ‘Tough Nature-Inspired Helicoidal Composites with Printing-Induced Voids’, Cell Reports Physical Science. Elsevier BV, 1(7), p. 100109. doi: 10.1016/j.xcrp.2020.100109.
Yuan, Y. et al. (2022) ‘Manipulating impact damage modes in composite laminates by helical pitch angle and ply thickness’, Engineering Fracture Mechanics. Elsevier Ltd, 265(December 2021), p. 108383. doi: 10.1016/j.engfracmech.2022.108383.
Zelazny, B. and Neville, A. C. (1972) ‘Quantitative studies on fibril orientation in beetle endocuticle’, Journal of Insect Physiology. Pergamon, 18(11), pp. 2095–2121. doi: 10.1016/0022-1910(72)90243-0.
Zhu, D., Ortega, C. F., et al. (2012) ‘Structure and Mechanical Performance of a “Modern” Fish Scale’, Advanced Engineering Materials, 14(4), pp. B185–B194. doi: 10.1002/adem.201180057.
Zhu, D., Szewciw, L., et al. (2012) ‘Structure and Mechanical Performance of Teleost Fish Scales’, MRS Proceedings, 1420, pp. mrsf11-1420-oo01-05. doi: 10.1557/opl.2012.503.
Zimmermann, E. A. et al. (2013) ‘Mechanical adaptability of the Bouligand-type structure in natural dermal armour’, Nature Communications. Nature Publishing Group, 4. doi: 10.1038/ncomms3634.
Artículos similares
- Diego Peña Lara, Daniel Suescún Díaz, Hernando Correa, Teoría de variable aleatoria aplicado a la transición de fases del sistema KI-AgI , Revista EIA: Vol. 22 Núm. 43 (2025): Tabla de contenido Revista EIA No. 43
También puede {advancedSearchLink} para este artículo.